The field of nuclear astrophysics started with basic questions regarding our Sun. It is obvious that life on Earth depends on nuclear processes deep inside the Sun, but how exactly the nuclear transmutations occur was not understood for some time. The breakthroughs came at the end of the 1930’s: Bethe and Critchfield uncovered a sequential reaction sequence fusing hydrogen (H) to helium (He), now referred to as the “pp1 chain”, while Bethe and von Weizsacker proposed a cyclic reaction sequence, now called the “CNO1 cycle”, that has the same end result of synthesizing He from H. For this early work, the Nobel Prize was awarded to Hans Bethe in 1967. It is interesting to point out that Bethe originally thought that the Sun derives most of its energy via the CNO1 cycle. Part of the problem was that some of the key nuclear reaction cross sections were poorly known. When more reliable cross sections could be estimated in the 1950’s, it became apparent that it is in fact the pp1 chain that governs the energy production in the Sun. The important lesson is that accurate nuclear physics information is crucial for our understanding of stars.
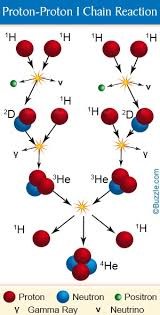
Some obvious questions followed immediately: how do other stars produce energy? How do they evolve and why do some of them explode? And perhaps the key question: where were the elements found on Earth produced? They were certainly not produced inside the Sun and, therefore, other processes are required to explain their origin. In this regard the solar system abundance distribution of the nuclides became of paramount importance. It demonstrates a rather complicated structure. The different processes giving rise to the observed features were explained by Burbidge, Burbidge, Folwer and Hoyle and by Cameron. These papers laid the foundation of the modern theory of nuclear astrophysics. For this work, the Nobel Prize was awarded to Willy Fowler in 1983.
Briefly, H and He are the most abundant elements and are made in the Big Bang. The abundance curve then drops sharply by 8 orders of magnitude. The species Li, Be and B are so quickly destroyed inside stars that their production must take place elsewhere. In fact, they are believed to be produced by cosmic-ray spallation. The abundance curve increases sharply at C and O. These are the most abundant elements after H and He and, incidentally, are the species life on Earth is based on. For increasing mass number the abundance curve decreases, but then produces a maximum near Fe, Co and Ni, referred to as the iron peak. Interestingly, these nuclides exhibit the largest binding energies per nucleon. So far, most of the species have been produced by nuclear reactions involving charged particles. To explain the origin of the nuclides located beyond the iron peak, however, fundamentally different processes are required. Those species are mainly produced via the capture of neutrons by the s-process and the r-process.
In this lecture, we will focus on charged particle processes important for stellar nucleosynthesis and energy production in stars. It will become apparent how quantum mechanical processes govern the evolution of large-scale objects in the Universe. This inter-connection is fascinating and remarkable, especially in view of the complex interplay of nuclear physics, hydrodynamics, atomic physics and plasma physics in stars. We may state without exaggerating that after several decades of research, stellar evolution and nucleosynthesis are among the most successful theories humans possess. The account given here is based on a recently published book, to which the student is referred for more detailed information.
The single most important that determines the evolutionary fate of a star is its mass. The larger the mass, the larger is stellar property the temperature and pressure in the core. Thus nuclear energy must be generated at a faster pace in order to stabilize the star, implying a larger luminosity and faster fuel consumption. Consequently, the larger is the mass the shorter the stellar lifetime. Before continuing, it is worthwhile to discuss briefly a few astrophysical phenomena, since they will be mentioned in the following. We start with globular clusters. These are located in a spherical space surrounding the Galactic center, called the halo. A typical cluster consists of 104-106 stars and is metal poor compared to the Sun, implying that it was formed during the early stages of Galactic evolution. The stars in a single cluster were formed around the same time from material of very similar composition.
REFERENCES
Transmutation – ядерное превращение, breakthrough – большое достижение, reaction sequence – последовательность реакций, pp 1 chain – протон-протонный цикл 1 или рр1 цикл, CNO1 cycle – цикл CNO1, cross section – сечение (ядерной реакции), cosmic ray spallation – расщепление космических лучей, abundance curve – кривая распределения (распространенности), iron peak – железный пик, binding energy – энергия связи, nucleon - нуклон, charged particles – заряженные частицы, s-process (slow-process) – с-процесс (медленный процесс), r-process (rapid-process) – р-процесс (быстрый процесс), stellar nucleosynthesis – звездный нуклеосинтез (т.е. синтез ядер внутри звезд), quantum mechanical processes – квантово-механические процессы (т.е. процессы, описываемые законами квантовой механики), stellar evolution – эволюция звезды, stellar property – характеристика звезды, luminosity – светимость (яркость), fuel consumption – потребление топлива, stellar lifetime – жизненный цикл звезды, globular clusters – сферическое скопление звезд, halo - гало, core - ядро, nuclide - нуклид